
The history of Thermoelectrics
In 1821, the phenomenon of thermoelectric was first discovered by Seebeck. In a thermoelectric material there are free electrons or holes which carry both charge and heat. To a first approximation, the electrons and holes in a thermoelectric semiconductor behave like a gas of charged particles. The gas molecules at the hot end will move faster than those at the cold end, so there will be a net build up of molecules (higher density) at the cold end. If the molecules are charged, the buildup of charge at the cold end will also produce a repulsive electrostatic force (and therefore electric potential) to push the charges back to the hot end. The electric potential (Voltage) produced by a temperature difference is known as the Seebeck effect.

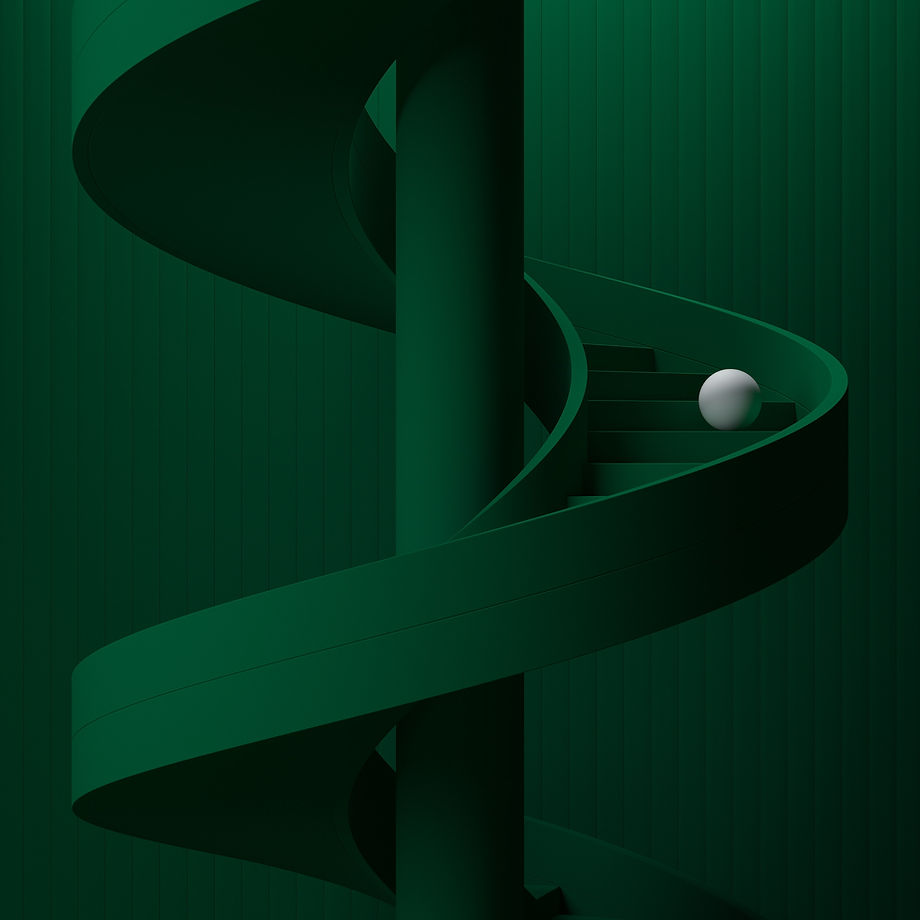
The figure shows a typical schematic for thermoelectric module composed by n-type and p-type semiconductors used for electrical generators and thermoelectric coolers. Taking the thermoelectric cooler as an example, as electrical current is injected into a closed loop, the direction of the electrical current is opposite with that of the electrons in n-type materials, and is the same with that of the holes in p-type material. Therefore, heat can be taken away at the joints of n-type semiconductor by the electrons which are pushed away by the injected electrical current and by holes in p-type semiconductor. Thus, the temperature at the joints is decreased as the electron current keeps injecting in the close loop. The phenomenon described above is the basic concept for thermoelectric cooler.
The state-of-art thermoelectric cooler comprises Bi2Te3-Sb2Te3 (p-type Bi0.5Sb1.5Te3 semiconductor) and Bi2Te3-Sb2Se3 (n-type Bi2Te2.7Se0.3 semiconductor), and both of them are considered as good thermoelectric materials with high figure-of-merit (zT=1 at room temperature). For a thermoelectric power generator, the most commonly used materials are PbTe and Si0.8Ge0.2, an the optimal temperature window for those two materials are at 900℃[88]. Nowadays there are many kinds of commercialized thermoelectric products such as thermoelectric cooler, small and portable refrigerator, beverage cooler powered by USB, etc.
Another application, thermoelectric generators, is widely used for transportation, industry and civil use, especially in waste-heat-recovery. For example, the Department of the New Energy and Industrial Technology Development Organization (NEDO) in Japan has devoted 150 million yen within two years in waste-heat-recovery by developing the high-efficiency thermoelectric material. Another case comes to the automobile used in daily life. The waste heat produced in automobile engines is considerable; only 25% of the fossil-fuel energy is converted in driving the engine, while 5% in frictional loss and 30% in engine-cooling. About 40% of fuel transformed into waste heat and released to the environment. The waste heat in automobile engines not only worsens the greenhouse effect but consumes the precious fossil-fuel energy. The conception for green-car by introducing the thermoelectric generator (TEG) in engine is design by BMW in 2009. Under normal driving speed, this TEG can provide 250 watts electricity and, in the meantime, reduce about 2% of CO2 emission.
In summary, although the task in seeking the prospect thermoelectric materials in replacement of other energy resources such as fossil fuel, wind power, water power, etc., still remains challenging. Thermoelectric materials still exhibit great strengths such as portable, no moving part, high reliability and environmental-friendly. Thermoelectric materials are benefit to our future life and are worth of furthering study.
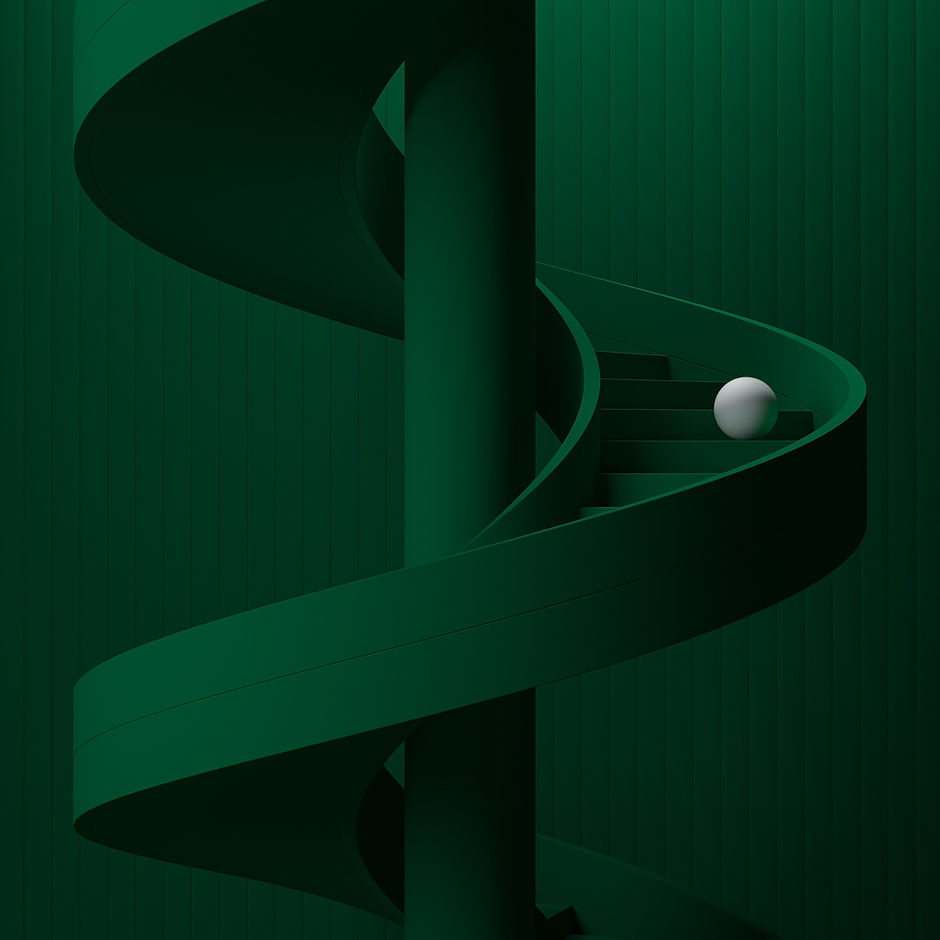
Bulk nanostructured thermoelectric materials
Thermoelectric materials had been struggled in breaking the bottleneck of improving the figure-of-merit larger than unity in 1960s-2000s. The discovery and devolvement of nanostructured TE materials provide the solution, and improving the zT greater than two. Two possible approaches come out to improve the figure-of-merit; the first one is by using materials with complex crystal structure such as skutterudites, clathrates, and Zintl phases which lead to low lattice thermal conductivity. The second approach, which is also focused on this study, is by introducing nanostructures into bulk thermoelectric materials. The general notion is that the ideal nanostructures can decrease the lattice thermal conductivity while minimally dispute the electronic transport property. In 1993, Hicks and Dresselhaus first proposed that quantum confinement of electrons and holes would increase the power factor S2ϭ independently, resulting in the figure-of-merit larger than unity.
Research on reducing lattice thermal conductivity have been intensively pursued, due to the capability of enhancing phonon-scattering through material engineering, such as grain size reduction, grain alignment along the transport direction, nanocomposite formation, etc.. Nanostructuring in TE material has been considered as an effective way of reducing thermal conductivity while minimizing the decrease of electronic mobility. Embedded nanosized phases that created extra interfaces with submicron spacing length are capable of scattering the phonons with mid-to-long mean-free-path. The advantage of nanostructure in reducing the thermal conductivity below the alloys’ nature properties can be illustrated in the figure. As shown in the left figure (a hypothetic atomic-scale image), the atomic defects, grain boundaries, and nanoparticle(or nanodots, superlattice, etc.) provide lots of scattering centers, and, subsequently the phonon scattering is enhanced. (Ref. C. J. Vineis, et al.,” Nanostructured Thermoelectrics: Big Efficiency Gains from Small Features.” Adv. Mater., Vol. 22, pp.3970-80, (2010)
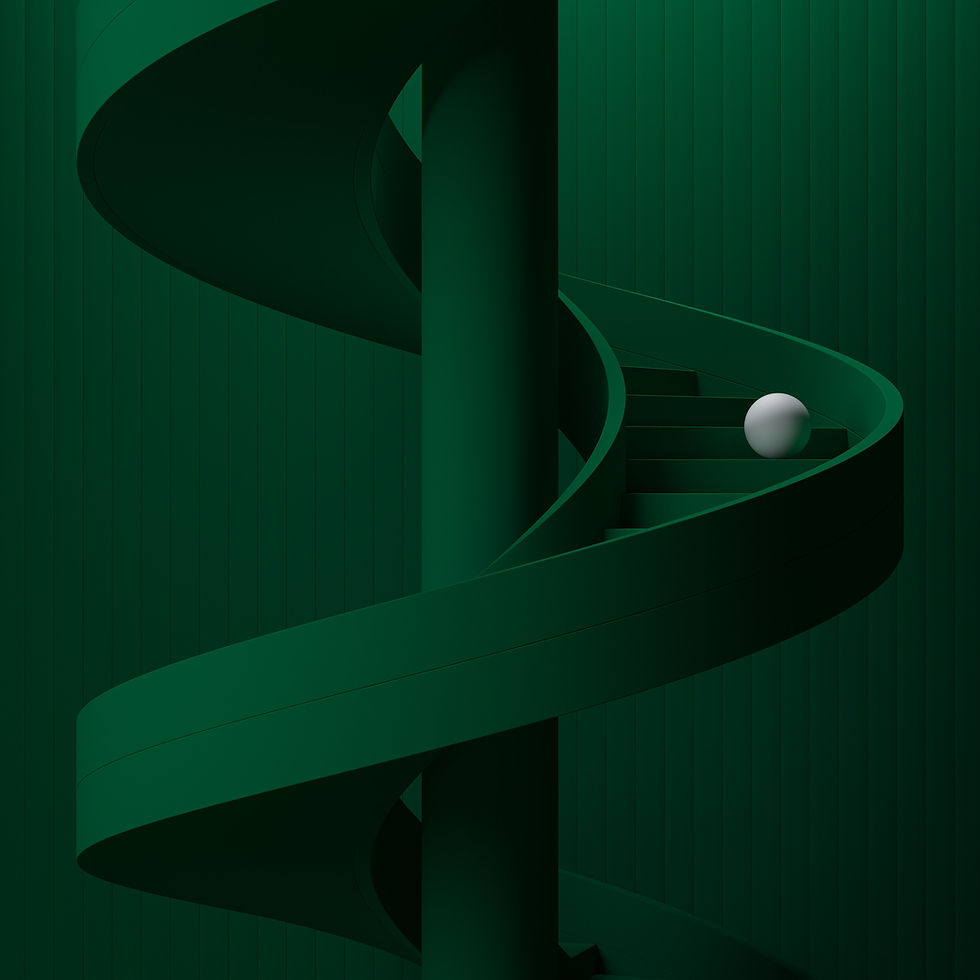

Efficiency is proportional to a zT value which composed of Seebeck coefficient, electrical resistivity and thermal conductivity. Three parameters are conflicting with each other. For example, it requires small carrier concentration and large effective mass to achieve high Seebeck value; however, for electrical resistivity, it requires large carrier concentration and large mobility. When it comes to lower the thermal conductivity, it needs small carrier concentration. Thereofore, our biggest challenge is to find out an optimal carrier concentration that leads to the highest zT. Based on the zT peak values, TE materials can be classified into three temperature ranges : low-temperature(T<400K), mid-temperature(400K-800K) and high-temperature (T>800K). The material system we are interested is in the mid-temperature region, with the best potential for waste heat recovery. Material systems suitable for the mid-temperature region include PbTe and LAST.
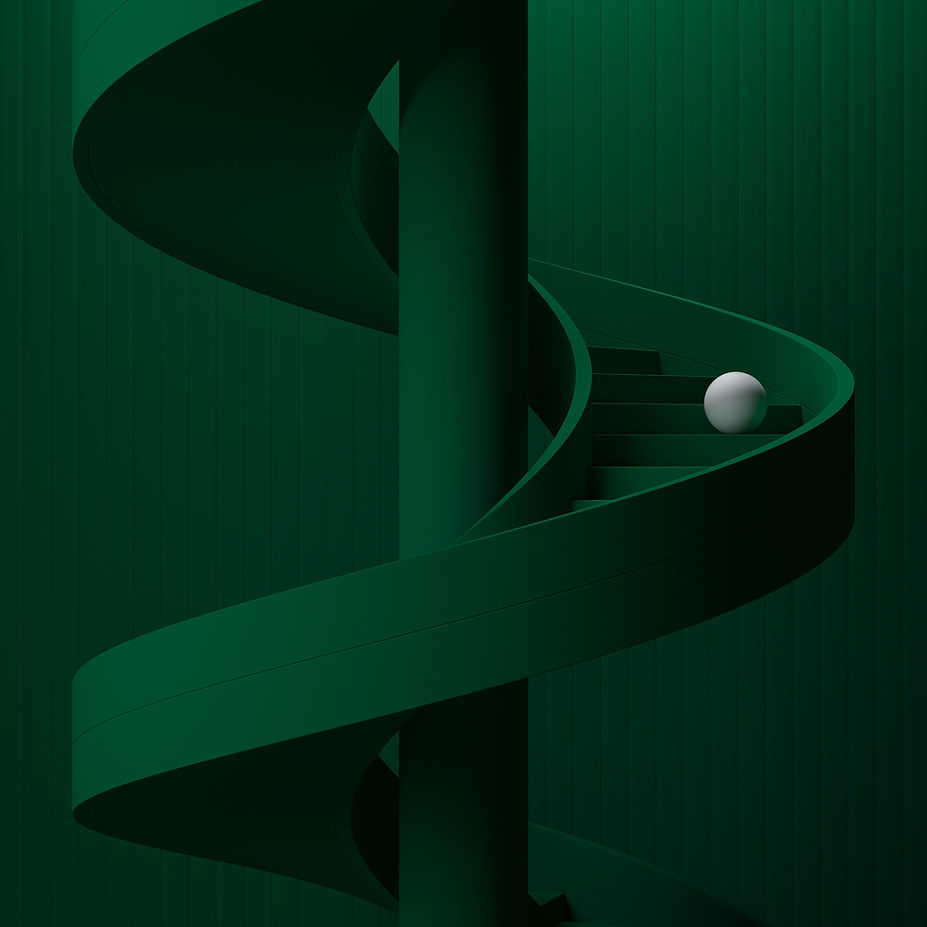
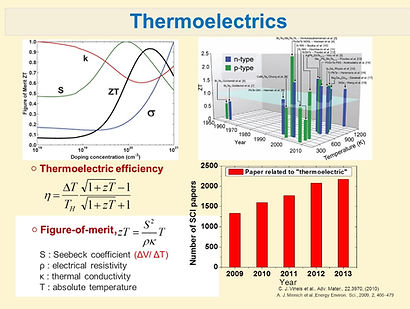
Therefore, thermoelectric materials can convert heat to electricity reversibly. The heat-to-electricity converting efficiency is related to the Carrot efficiency, and also the figure-of-merit (zT). Figure of merit is comprised of three different materials' physical parameters, which are the Seebeckcoefficient, the thermal conductivity and the electrical resistivity. So it can be imaged that a good thermoelectric materials should exhibit high Seebeckcoefficient and low electrical resistivity and low thermal conductivity. In 1960s, the zT value of Bi2Te3 was around one; however, for the next 40 years, the research area of thermoelectric faced a bottleneck until the development of nanotechnology; after 2000, the zT values could reach above two thanks to the development of nanostrcutured bulk materials. Also, the publication number in this specific area steadily increases in recent years. For example, in 2012, there are more than 1600 journal papers entitled thermoelectric being published.
Thermoelectrics is very important, especially in the field of waste heat recovery. The energy flow chart of the U.S. shows that more than 60% of the energy was wasted, and most of it in the form of waste heat. Thermoelectric materials, which allow directly conversion between thermal and electrical energy, can therefore recover the lost energy. Despite thermoelectrics exhibits lots of advantages, the biggest challenge and effort are focused on improving the figure of merit.
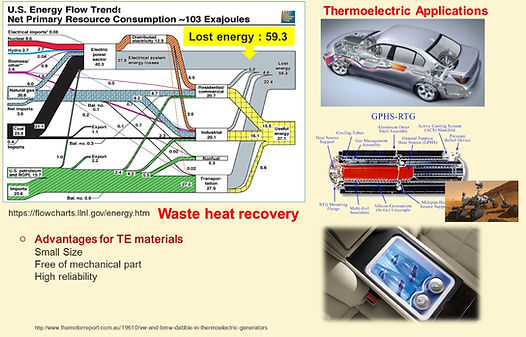

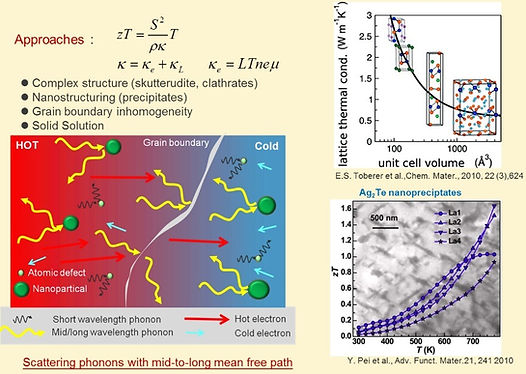